Science of Rare Earth Elements
Introduction
The rare earth elements are 17 metallic elements found in the middle of the periodic table. Some of the rare earth elements have unusual magnetic, luminescent, and electrical properties that make them valuable for industrial applications and in manufactured products. But because these elements have a very similar electron structure (as explained in “Producing the Rare Earth Metals” below), separating them from each other is difficult. Although mineral ores containing rare earths can be found on every continent, most production of rare earths today happens in China.
Rare Earth Element Properties
What Gives Rare Earth Elements Their Useful Properties?
Adam Schwartz (Director, Ames Laboratory):
So what makes rare earths remarkable for optical properties, electronic properties, magnetic properties are those 4F electrons and the way those 4F electrons interact with each other and with other elements when the rare earths are mixed in. For example, the most powerful magnet in the world, neodymium-iron-boron magnets, only have 2 atoms of neodymium, 17 atoms of iron, and 1 atom of boron. So neodymium is not even the primary element in that compound. However, just having those 2 atoms of neodymium creates the best properties of any magnetic material out there.
Eric Schelter (Director, Center for the Sustainable Separations of Metals):
Rare earths are really useful for storing and manipulating and using quantum information because of their fundamental characteristics, many of which are unlike any other element in the periodic table. And so if you want to sort of manipulate quantum information, you need some kind of a quantum mechanical property, and one of the obvious ones that people use is spin. So spin is an inherent quantum mechanical property of fundamental particles. So electrons have a spin, for example, and protons and a nucleus—with the ensemble of protons and neutrons—also have a nuclear spin. They’re two different flavors of this quantum mechanical property, and it is that fundamental quantum mechanical property which is essential to be able to manipulate in order to work in quantum information. It’s a totally different way of controlling and manipulating information and doing things like computation, which nature uses on this atomic scale. And so rare earths are really good at this because of their characteristics, for example, associated with their spin. One of the big challenges for developing quantum information and turning it into a viable technology is that it doesn’t last very long. It’s a very sensitive type of state to have these different spins of fundamental particles to interact with one another and to be manipulated, to ask them a question and then have them present an answer. And so that state, that ability to do sort of logic operations associated with computings, that ability to put them into a configuration or into a state where questions can be posed in a logical sense, is hard to maintain. Because it’s very delicate. So temperature, you know, different thermal variations, will totally destroy this special quantum state that we need to be able to do quantum information processing. So rare earths have intrinsic atomic and electronic properties, that is, the properties of their fundamental particles, which puts them into states that can survive long enough to ask these quantum computational questions.
Credits: The Rare Earth Elements Project is made possible by a generous grant from Roy Eddleman, founder of Spectrum LifeSciences.
Illustrations and animations: Claud Li
Music: “Massive Attack” by Podington Bear
(c) 2020 Science History Institute
Magnetic Properties
Includes neodymium (Nd), dysprosium (Dy), and samarium (Sm)
These rare earth elements are particularly valued for their magnetic properties. Magnetic fields are generated by unpaired electrons aligned so they spin in the same direction. The orbital electron structure of these elements contains many unpaired electrons, which means these rare earth materials can store large amounts of magnetic energy. A neodymium magnet can store about 18 times more magnetic energy than an iron magnet of the same volume. Rare earth magnets are widely used today in many applications, including wind turbines, electric motors, guidance systems on aircraft and missiles, speakers for personal electronics, and computer hard drives.
Unfortunately, in their pure form these rare earth elements lose their magnetism before they reach room temperature. To ensure that these rare earth metals retain their magnetism, they are combined with a transition metal, such as iron or cobalt. The resulting metal alloys display great magnetic strength and retain their magnetism at higher temperatures. Examples of these alloys are neodymium-iron-boron magnets (Nd2Fe14B) and samarium-cobalt (SmCo5) magnets. Neodymium alloys are quite brittle, however, and need to be coated with other metals to produce magnets that do not break apart under ordinary use. The need to remove these coatings increases the cost of recycling rare earth magnets.
Luminescent Properties
Includes europium (Eu), yttrium (Y), erbium (Er), and neodymium (Nd)
Some of the rare earth elements have luminescent properties, meaning they give off light when stimulated by electromagnetic radiation. These elements are used as phosphors (light-emitting substances) in energy-efficient light sources, such as light-emitting diodes (LEDs) and compact fluorescent light bulbs. Europium phosphors produce red light that proved crucial to the development of color television in the 1960s. The fluorescent properties of erbium ions make them especially useful for amplifying signals transmitted through glass fibers. Erbium-doped fiber amplifiers intensify the signal in high-capacity fiber-optic lines that carry internet data and long-distance telephone calls around the world. (In materials science “doping” means adding an element in very small concentrations—less than 1,000 parts-per-million—to alter a substance’s electrical or optical properties.) Lasers represent another use of the luminescent properties of various rare earth elements. Yttrium-aluminum-garnet (YAG) is a common medium for solid-state lasers. When doped with erbium, YAG lasers produce wavelengths of focused light useful for oral surgery and dentistry. When doped with neodymium, YAG lasers are widely used in manufacturing, medicine, and military applications, such as range finding (determining the distance to the target) and target designators (pinpointing the target) for guided missiles.
Electrical Properties
Includes cerium (Ce), lanthanum (La), neodymium (Nd), and praseodymium (Pr)
The electrical properties of rare earth elements make them useful for nickel–metal hydride (NiMH) batteries. The anodes of these batteries are made of mischmetal, a mixture of cerium, lanthanum, neodymium, and praseodymium that is cheaper to make because the rare earth metals do not have to be fully separated from each other. The rare earths give the battery a higher energy density (greater capacity to store energy in a given weight) and a better ability to retain capacity after many discharge-recharge cycles. These batteries are widely used in hybrid cars and portable construction tools.
Catalytic Properties
Includes cerium (Ce) and lanthanum (La)
The electron structure of the rare earths makes them useful as catalysts (accelerators) for chemical reactions. Cerium and lanthanum are the main rare earths used in catalytic applications, since these two metals are much more abundant and sell for much lower prices. Cerium is used in the catalytic converters on gasoline-powered cars. This element readily captures and gives up oxygen, making it useful for converting poisonous carbon monoxide (CO) into nontoxic carbon dioxide (CO2) via oxidization. Lanthanum is commonly used in fluid catalytic cracking, the process of refining crude oil into specific useful hydrocarbons such as gasoline.
The Geology and Geography of Rare Earth Elements
The rare earth elements are not especially rare from a geological perspective. The rare earth elements occur at very low concentrations in most massive rock formations, and sources of the rare earth elements have been found on every continent as well as on the ocean floor. The geological challenge is to find ores with sufficient concentrations of rare earths to make it economically feasible to mine and process them.
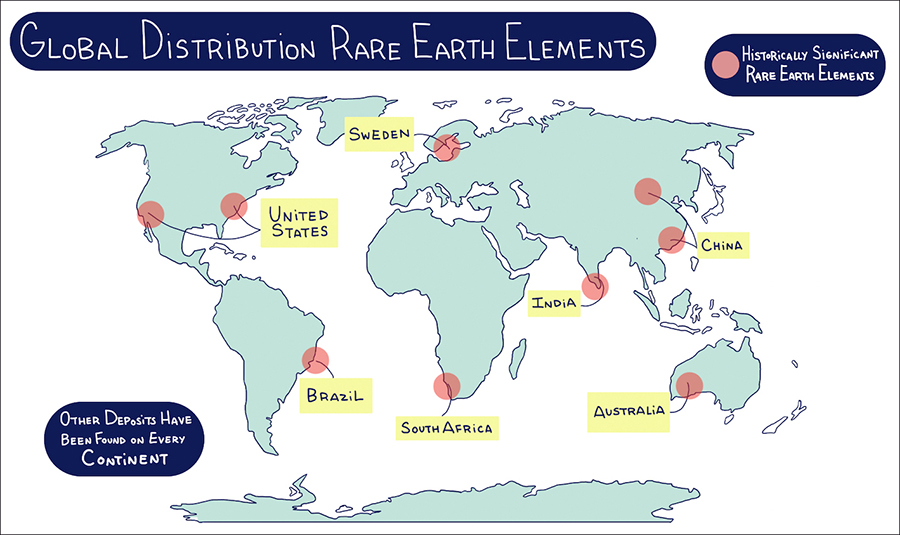
Rare earth elements are found on every continent but not always in great enough concentrations to make mining worthwhile.
For economic reasons most production historically has involved mining one of three minerals that contain higher proportions of the rare earth elements: monazite, bastnaesite, and rare earth–bearing (ion-adsorption) clays. Each deposit of these minerals, however, contains different proportions of the individual rare earth elements.
Scientists divide the rare earth elements into two categories—light and heavy—but opinions vary on exactly where the heavy elements begin. Essentially, the heavy rare earths are much less abundant than the light rare earths, and so the heavy ones sell for much higher prices. The value of an ore is thus determined largely by the proportion of heavy rare earths found within it.
Monazite was the primary mineral mined to produce rare earth elements from the 1890s until the 1950s. Although the composition of monazite ore varies, the concentration of rare earth elements is usually quite high as compared with other ores, but one disadvantage of monazite is that it also contains a much higher percentage of radioactive thorium. For many years monazite deposits were mined in North Carolina and in Brazil and India. In the 1950s South Africa briefly became the world’s leading exporter of rare earth ores. Today monazite is a relatively small source of rare earth element production—mined primarily at the Mountain Weld Mine in Australia and shipped to Malaysia for separation and processing.
Beginning around 1960 bastnaesite became the dominant mineral mined for rare earths, as concern grew about thorium and radioactivity. Bastnaesite typically contains light rare earth elements, with smaller amounts of the heavy elements. Bastnaesite has much less radioactive thorium than monazite but does contain large quantities of carbonate fluoride (CFO3). The fluoride released during mining can cause bone defects and other human health problems if it is not controlled. Bastnaesite from the Mountain Pass Mine in California became the main source for rare earth elements used in the United States and circulated in global trade from 1960 through the 1990s. China exploited rich bastnaesite deposits at its Bayan Obo Mine in Inner Mongolia as it increased its rare earth production beginning in the 1970s.
In 1970 ion-adsorption clays were discovered in scattered locations across seven provinces in southern China. These clays have an exceptionally high proportion of heavy rare earth elements. As deposit-rich granite rocks erode, dissolved rare earth elements are deposited as a thin film onto clay in the top layers of small mountains. This process naturally separates the rare earths from thorium and uranium, producing ore with significant rare earth concentrations. Today these deposits account for a large percentage of the world’s total production of heavy rare earth elements.
Producing the Rare Earth Metals
The difficulty of separating and purifying the rare earth elements makes their production very expensive. Mining of rare earth metals, as with all metals, begins by digging rock or sand from the ground, separating out the valuable ore from the waste rock (tailings), and then refining the ore to separate out the metal. But producing the rare earth metals, unlike other metals, requires an extensive additional stage where the various rare earths are separated from each other.
This final stage of production is difficult and costly. The atoms in rare earth elements all display very similar chemical behavior as a consequence of their peculiar atomic structure. The chemical properties of an atom are largely determined by its valence, or bonding, electrons. These electrons are usually in the atom’s outermost electron shell, and most elements next to each other in the periodic table have quite different chemical properties. (Think of explosive hydrogen, which appears on the periodic table next to the nonreactive noble gas helium.) However, for the rare earth elements the outermost electron shell is filled the same way, causing these elements to react in similar ways. The similar reactivity is what makes it so hard to separate them from one another.
To overcome this problem, metallurgists use a variety of complex separations processes. For commercial use the most common of these is a lengthy process called solvent extraction. In this process rare earths are dissolved at different concentrations in two liquids that are immiscible (naturally separate when allowed to settle, like oil and water). By repeatedly mixing various acids that have affinities for (have a tendency to react with) different rare earth elements and then allowing these mixtures to settle, metallurgists gradually achieve higher concentrations of specific metals in each separation. Repeated over hundreds or thousands of cycles, this process can achieve purities higher than 99.9%.
How Are Rare Earth Elements Separated Today?
Eric Schelter (Director, Center for the Sustainable Separations of Metals):
So rare earths today across the world are separated using one process, and that’s called solvent extraction. Actually, many different metals are purified in this way, and many different materials are purified in this way using solvent extraction. So solvent extraction works according to principles of chemical thermodynamics.
I’ll walk you through sort of the general idea here. So basically you have a mixture of two liquids that don’t mix with one another. They kind of remind me of salad dressing, like vinegar and oil that form that sort of dispersion. And so if you have rare earths that are loaded into the vinegar phase, into the water phase, you can extract them. You can separate them into the oily phase through this process with an extractant, which is basically a compound that you would add that would move the ions from one phase into the other. And so every time you do that, you end up with a slightly different concentration, or population, of your mixture in the two phases. So at the beginning we might have 50/50 of two different rare earths dissolved in the acid phase, in the vinegar phase. And then when we do the extraction by contacting those two liquids together that don’t mix, with our extractant involved, then we move some portion of one of the rare earths into the oily phase. But at the end of the day we might have a 60/40 population in one phase versus the 50/50 that we started with in the other phase. So it’s a very inefficient process, and in fact the selectivity is very low: 60/40 would be great in terms of the populations, but it never works that well. And so that means that we have to extract and back-extract over and over again thousands and thousands of times in order to get a purified phase. So basically you’re just slightly enriching, you’re slightly purifying a given rare earth when you go through this process, and it requires a lot of time and circuits of solvent moving around and contacting with each other in order to really purify a material out to 99%, which would be the goal.
And so solvent extraction works really well, and chemical engineers have developed it into a highly scalable and effective process, but on a single pass it’s really inefficient. And so this is the reason why rare earth production produces a huge amount of waste. It’s because you have to do this process so many times in order to really purify the individual elements.
Credits: The Rare Earth Elements Project is made possible by a generous grant from Roy Eddleman, founder of Spectrum LifeSciences.
Illustrations and animations: Claud Li
Music: “Caterpillar Brigade” by Podington Bear
(c) 2020 Science History Institute
Unfortunately, solvent extraction is slow, energy-intensive, expensive, and supremely difficult to calibrate (adjust) with the high precision necessary for success. Every geological deposit of rare earths is slightly different, which makes solvent extraction hard to optimize (to make as efficient as possible). As a result, producers that have been using the same ore sources for a long time have a significant economic advantage in the marketplace: over time they have already found the most efficient separation process for that ore.
Potential Alternative Sources of the Rare Earth Elements
Researchers are exploring a variety of new ways to produce rare earth elements.
Phytomining
Phytomining may offer an alternative, especially for the light rare earth elements. Some plants selectively take up these elements from the soil and store them in their tissues. Various plants, such as sunflowers, canary grass, and some ferns, can be used to accumulate certain metals without digging new mines. The crops can be planted in many kinds of soils and can be harvested to extract the metals. After the plant material is fermented (producing biogas that can be burned for energy), the rare earth metals can be separated using solvent extraction. While phytomining has been used since the 1990s to remove heavy metals, such as cadmium and lead, from polluted soils, it is not currently used for commercial rare earth production.
Extraction from Mining and Commercial Waste
The material we currently classify as waste (runoff, tailings, old electronics) could be repurposed to serve as potential new sources of rare earth elements.
Scientists at the West Virginia Water Research Institute, funded by the U.S. Department of Energy, have been studying ways to extract rare earth elements from the water that drains from abandoned mines. The water draining from mines is currently an expensive liability because of its toxicity, but deciding to extract valuable resources from it would ensure the water is treated and make it an asset to the clean energy economy.
Phosphogypsum, a weakly radioactive waste material from the production of phosphate fertilizers, also contains rare earth elements and has been suggested as a potential future supply of these metals.
The vast “urban mine” of obsolete electronics and industrial goods offers another possible source through recycling. Extracting the metals from these sources, however, would still depend on current separation methods such as solvent extraction.
Alternative Separations Processes
Alternatives to solvent extraction are also being researched. Eric Schelter, a specialist in advanced rare earths separations chemistry at the University of Pennsylvania, has developed techniques for using ligands (ions that selectively bind to particular atoms) to separate neodymium and dysprosium from each other at room temperature using common lab equipment. Researchers at Ames Laboratory have developed another alternative, an acid-free technique for dissolving and recovering rare earths from shredded computer hard drives.
None of these techniques can currently produce rare earth elements in significant enough quantities or at prices low enough to compete with production in China, where solvent extraction is used. However, the economic situation could shift with any future changes in supply, demand, regulation, and the willingness of manufacturers and consumers to pay more for materials from alternative sources.
How Can Chemistry Contribute to Rare Earth Element Sustainability?
Eric Schelter (Director, Center for the Sustainable Separations of Metals):
So chemistry and sustainability are really strongly tied together. I mean, chemical industry and all of the products around us every day are the result of that chemical industry. And so how we get stuff and how we do it sustainably is a really important question for the whole field of chemistry right now. And this ranges from microplastics in the ocean and processing those materials, to coming up with new plastics that can be depolymerized so they don’t persist in the environment forever, to ethical and sustainable production of raw materials like metals. Chemistry has always been about getting stuff, in my opinion. I mean going back to Chilean saltpeter to make gunpowder and the development of the Haber-Bosch process, which supplanted that source of those materials. [The Haber-Bosch process allowed the creation of synthetic components for gunpowder and fertilizer, making mining of naturally occurring saltpeter unnecessary.] So it’s always been about new technology emerging that gives access to new stuff. And so the twist on it in the next century will be, how can we provide new materials or provide materials that are essential for emerging industries without causing so much environmental harm?
Credits: The Rare Earth Elements Project is made possible by a generous grant from Roy Eddleman, founder of Spectrum LifeSciences.
Illustrations and animations: Claud L
Music: “Big Poppa” by Podington Bear
(c) 2020 Science History Institute