When Carey Lea arrived at his summer house in 1886, his silver was the wrong color. Or at least some of it was.
Lea had been studying silver for decades. He was fascinated by the chemistry of photography, and silver solutions were essential to capturing images. Recently Lea had developed a new kind of silver colloid. It started off gold and changed to white after reacting with light. He called it “allotropic silver,” and he had brought three samples of it with him to his summer house.
One sample had been spread on paper, another spread on glass plates, while the third was loosely packed in glass tubes. All had been golden at the start of the jouncing, 600-mile trip by train and steamboat. But on arrival, the silver stored in the tubes had turned white without ever having been exposed to light.
Lea pondered this curious transformation. Some sort of mechanical force must be the culprit, he thought. It wouldn’t have been the bumps and bounces from riding the rails, otherwise all the samples would have been affected. Rather, he surmised, the friction of the particles rubbing against each other had initiated the chemical change.
He tested his hypothesis by packing up three more samples and sending them on a 2,400-mile train trip. When the shipment returned, Lea was proven right—the two loosely packed controls had turned white, while a sample tightly packed with cotton wool retained its original gold color.
This simple experiment was the first of a series of insights Lea would have into the ways mechanical force can initiate chemical reactions. More importantly, he would go on to reveal that mechanical force can spawn unique reactions and products. A three-year burst of research would establish mechanochemistry as a stand-alone field and Lea as its father.
But Lea was a father lacking heirs. He was a throwback to another time, a wealthy and reclusive gentleman scientist chasing curiosity in a private lab. When he died in 1897—just a few years after publishing his foundational work on mechanochemistry—the growth of the American chemical industry and chemistry as a trade and profession had ushered out the last of the gentleman scientists. Lea’s discoveries languished.
In recent decades, though, researchers have started to revisit mechanochemistry. In it they see the promise of a greener chemistry that substitutes mechanical force for heat and solvents. With renewed interest in mechanochemistry, Lea has also undergone a minor historical renaissance.
Matthew Carey Lea was born in 1823 into a remarkably wealthy and intellectual Philadelphia family. (The recorded spelling of Lea’s first name varies, but he went by Carey.)
His grandfather Mathew Carey was a publisher and political operator who rubbed shoulders with Benjamin Franklin and Thomas Jefferson. His father, Isaac Lea, uncle Henry Carey, and brother, Henry Lea, built the family business into one of the country’s biggest publishers.
But the family’s accomplishments extended much further. Lea’s uncle was an influential economist who left the business to advise President Abraham Lincoln. Lea’s father was an equally gifted conchologist who led several national science organizations after retiring. His brother became a well-known bibliophile and historian of the medieval period and Spanish Inquisition.
“The intellectual climate of the Carey and Lea households must have been formidable,” historian Edward Peters remarked.
But that climate took a toll on Carey Lea and his brother. Both suffered from spells of exhaustion and the manifestations of mental strain. While Henry managed his illness and went on to lead an active professional and civic life, Carey Lea receded from public view. Illness forced him to abandon a career as an attorney before it ever began. Instead, he escaped to Europe in search of “permanent relief, but without success,” noted George Barker in his biographical memoir of Lea.
After returning to Philadelphia, Lea entered the lab of chemist James Booth, with whom he had studied chemistry as a child. After honing his analytical abilities with Booth, he retreated to his home in the posh bedroom community of Chestnut Hill, where he had built a private lab.
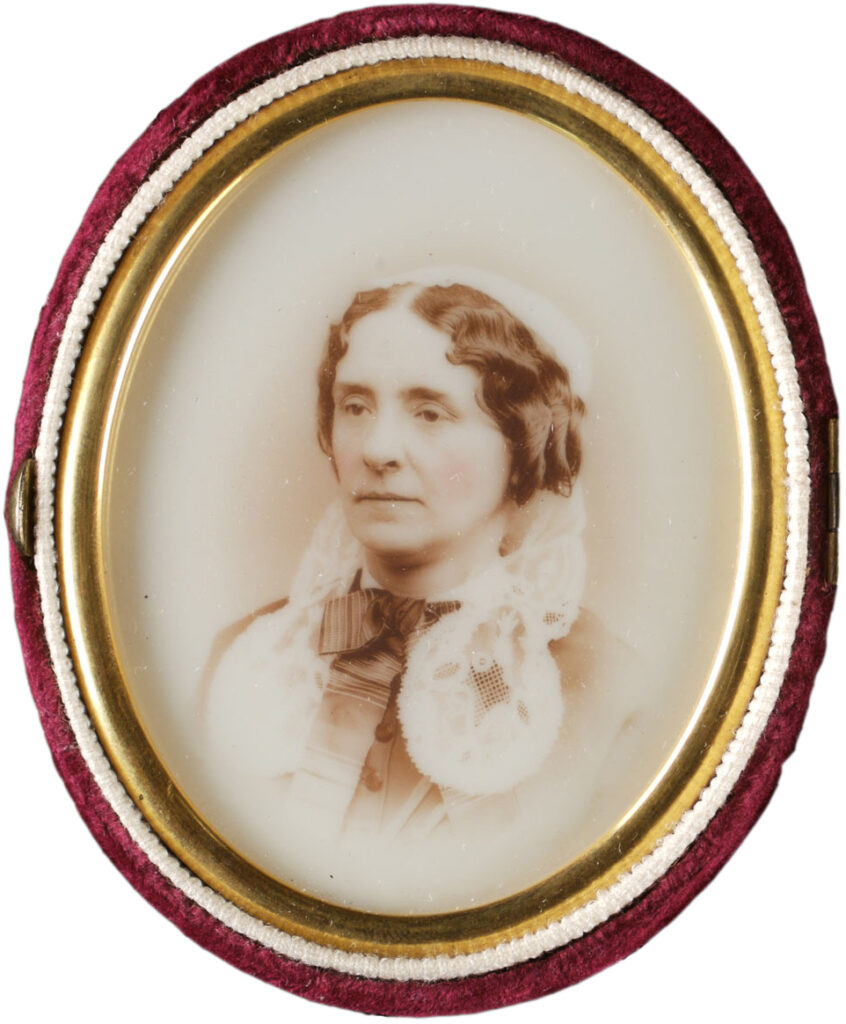
He married a widowed cousin (the sister of his brother’s wife), with whom he had a family. He married again after his first wife’s death. He kept a few close friends, his brother chief among them, but otherwise led a reclusive life spent inside his well-appointed lab. He rarely, if ever, presented his findings. He simply worked.
“Few chemists knew Lea personally,” wrote mechanochemist and historian Laszlo Takacs. “His weak health and a laboratory accident that damaged one of his eyes made him an elusive figure. He worked quietly and independently in his laboratory, keeping contact with the rest of the scientific community through publications.”
But if Lea’s public life seemed dull, his inner life was vibrant.
Lea’s Chestnut Hill lab was possibly the best private chemistry lab in the country. It must have been a sea of glassware, filled with rich colors that revealed the nature of chemicals. A set of experiments on separating platinum metals in 1864 resulted in a solution that was “magnificent red-purple” at high concentration, shading down through “a fine rose color,” and ending at “a still paler but unmistakable rose tint” at a concentration of one part in 100,000.
Color was an obsession throughout his career. In 1895 he proposed a rival system to Mendeleev’s periodic table that paired color with atomic weight as attributes for organizing the elements.
But the color Lea’s most associated with is silver, the elemental basis for one of the most revolutionary technologies to emerge during his life: photography.
Lea began experimenting with photography as early as 1840, when, at age 17, he created a series of images of plants and shells from his father’s natural history collections for display at the American Philosophical Society.
By the 1860s, Lea’s sole focus was photography. In 1868 he published A Manual of Photography, which became a standard reference work for photographers and others trying to understand the art and science of the technology. In it Lea offered practical advice on exposure times and special techniques for taking landscape and portrait images. It included chemical recipes for making the light-sensitive emulsions that photographers spread across glass plates and the chemical processes used to develop them. Innovatively, he described ways that the light-sensitive chemicals silver chloride and silver bromide could be used to generate different shades of color from metallic silver. (Figuring out how to fix these color images would take chemists another five decades.)
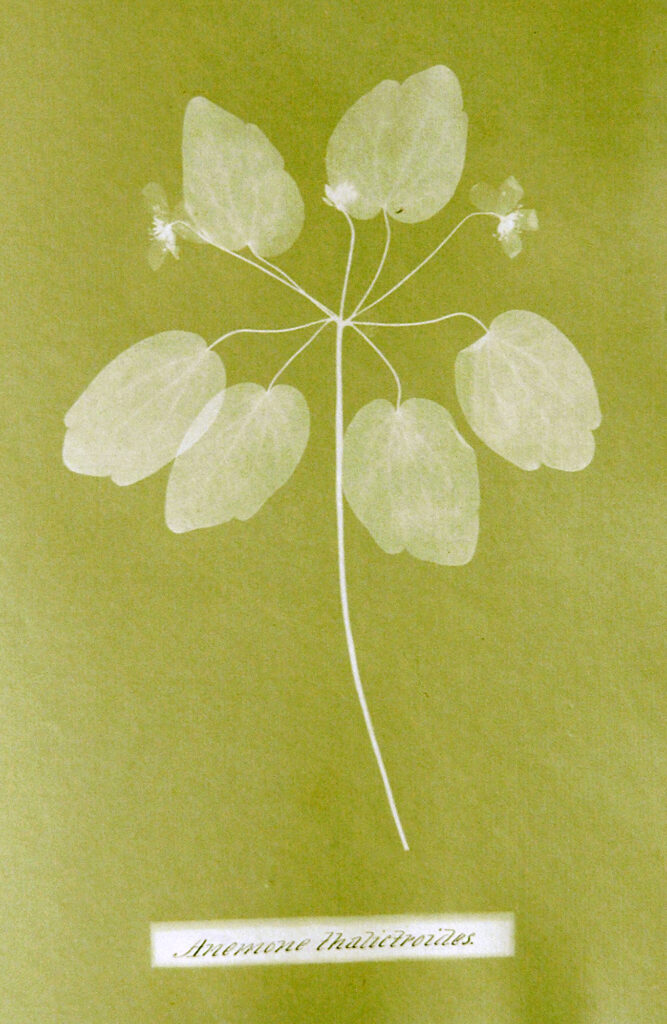
Lea’s Manual was one of the first purchases George Eastman made when he set out to make photography something anyone could take part in.
Photography at the time was an altogether messy and burdensome affair. It required dragging around heavy equipment, glass plates, and a jumble of chemicals and other materials. Photographers were forever waiting (for their plates to be exposed) or rushing (to develop their plates before they dried). Shooting a picture outside a studio was a life spent in cramped, often impromptu darkrooms slopping around noxious emulsions, developers, and fixers. Eastman, a young banker and overwhelmed amateur photographer, wanted to put an end to this chaos. The chemistry was the key.
Eastman began experimenting with emulsions that could work as a dry photosensitive coating applied to rollable, paper-based film. He wrote Lea for advice in early 1879, though Lea’s response is now lost, if he ever sent one. Eastman eventually succeeded in creating a dry and transparent film, the heart of the Kodak camera he introduced in 1888. (“You press the button, we do the rest.”) Eastman’s process, not surprisingly, used a developing chemical described by Lea.
“Lea’s contributions to the infant field of photographic science were unparalleled in the 19th century, and in many ways he did for the science of photography what Mathew Brady did for the art,” wrote David Whitcomb, an expert of silver chemistry and photography. In all, Lea published nearly 300 scientific articles related to the chemistry of photography.
Silver was always the magic element in photographic chemistry, and no one knew its properties like Carey Lea. It was through his investigation of silver salts and colloids that Lea began thinking about the effects mechanical force can have on the chemistry.
Lea’s observation of wrong-colored silver in 1886 launched a careful study of silver halides and mechanical force, resulting in four papers that marked the first systematic investigation into mechanochemistry. (Silver halides are chemical compounds formed between atoms of silver and atoms of bromine, fluorine, chlorine, or iodine.)
At first, Lea spent about two years exploring how samples of his silver colloid (“allotropic silver”) could be transformed. He found that light and physical force could produce similar transformations. For example, pressing a glass rod across a photographic plate covered with silver colloid created developable images, just as if the plate had been exposed to light. If mechanical force could cause a photochemical effect, Lea wondered if it could also cause effects that were usually triggered by heat.
“Lea demonstrated that any form of energy, including mechanical, was indeed capable of disrupting silver halide molecules,” wrote Takacs. Lea described how he squished samples of three different compounds in a press, exposing the samples to 100,000 pounds of static pressure per square inch for 24 hours. A change in color clearly indicated that some of the silver atoms had broken away from the halides.
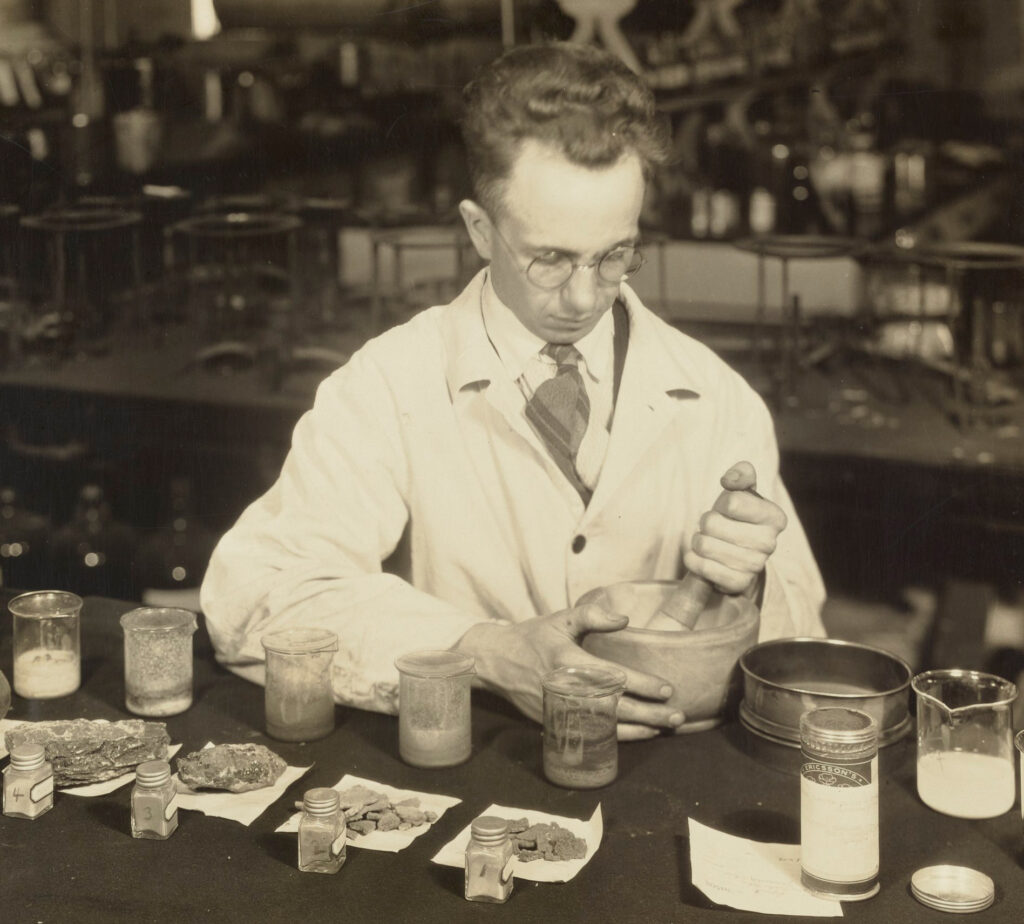
But shearing force was even more effective than static pressure. Lea used a handheld pestle to grind silver halides in a porcelain mortar. (Trituration is the technical term for this action.) After 10 minutes of shearing, discolored streaks began to appear in the silver chloride; the sample was largely decomposed after another 5 minutes of triturating.
During 1893 and 1894, Lea published three more papers that extended his investigations of mechanical force on chemical bonds. First, he cranked up his static press to see what happened when he squashed 15 materials known to change color when decomposed by light or heat. Several of these decomposed under static pressure, to varying degrees.
Lea’s next paper took aim at the common 19th-century dogma that chemical changes could not be brought about by “mechanical impulse.” Lea adopted a word coined by physical chemist Wilhelm Ostwald for this paper: mechanochemistry. Attacking at least 17 materials with his mortar and pestle, Lea showed that mechanical force could break down some materials just as heat would, sometimes more efficiently.
Even more significantly, Lea found that mechanochemistry could do things that weren’t possible with thermochemistry. For instance, heating mercuric chloride made it sublime (jump from solid to gas), but grinding it decomposed the molecules.
Taken together, his papers show that Lea was able to discriminate between the effects of heat and mechanical action while quantifying the results, work that made him “the true founder of mechanochemistry,” according to Takacs. “He not only showed that mechanical action was capable of inducing chemical changes, . . . he also proved that these changes were sometimes different from those produced by heat.”
But Lea’s insights came too late and from a place that was too far outside the chemical mainstream.
The social structure of chemistry had changed substantially over Lea’s lifetime. The influence of gentleman scientists, working in their personal labs, waned. Research, instead, flourished in labs backed by universities, government bureaus, and industrial companies. The expansion of universities created a growing population of credentialed chemists, who took salaried jobs in industry and government agencies. As chemistry grew, the chemical approaches that thrived were the ones recognized by this new generation of experts.
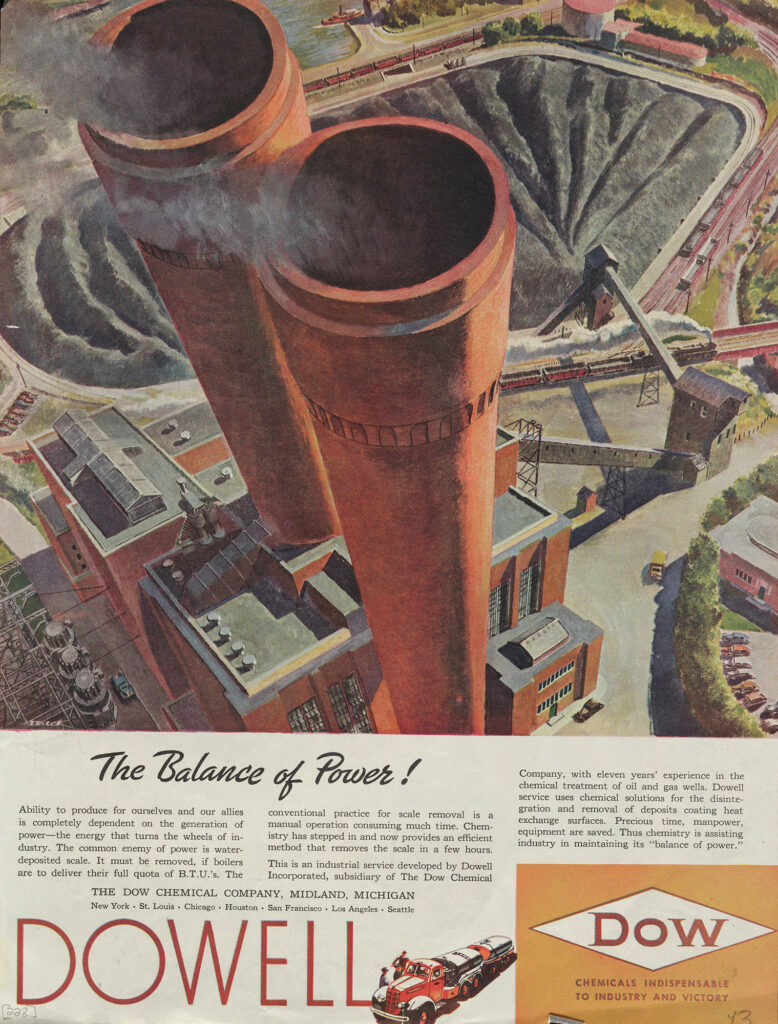
During the last decades of the 19th century, the German company Bayer industrialized invention and discovery in chemistry. Teams of research chemists, working with librarians and analysts, turned discoveries into salable products. Chemical engineers formed a new profession by scaling up the researchers’ laboratory processes for industrial production. Solvents and heat became familiar tools that both researchers and engineers successfully controlled to produce chemicals at industrial scale.
“It was this pattern of industrialized invention that became the international model for twentieth-century industry,” wrote William Brock in The Norton History of Chemistry. “Research was now a cooperative enterprise within a bureaucratic structure and in which a company, rather than a particular chemist, became the collective inventor.”
Mechanochemistry was largely ignored by the industry. Lea lacked the protégés and collaborators who could have integrated his insights into industrial-scale production. New chemical plants were designed by chemical engineers trained by solvent chemists working in university-based education programs.
By the time Lea died in 1897, the course of chemistry had been set, and mechanochemistry, the brainchild of a dedicated but solitary chemist, was left in its wake.
History is a tool wielded by the teller. We talk about historical figures in ways that advance our own ends. So it has been for the story of Carey Lea. At particular times and in particular places, his story has been constructed to further the pursuits of others.
Edgar Fahs Smith was the first to find something useful in Lea’s story. In 1923 Smith published a collection of biographies featuring Lea and other American chemists.
Smith built Lea into a hero, part of his “American Heritage” of chemical researchers who might inspire students to stay in the United States rather than pursue advanced education in Germany. Lea’s status as a Philadelphia-based researcher was particularly important to Smith in building his collection of chemical supermen:
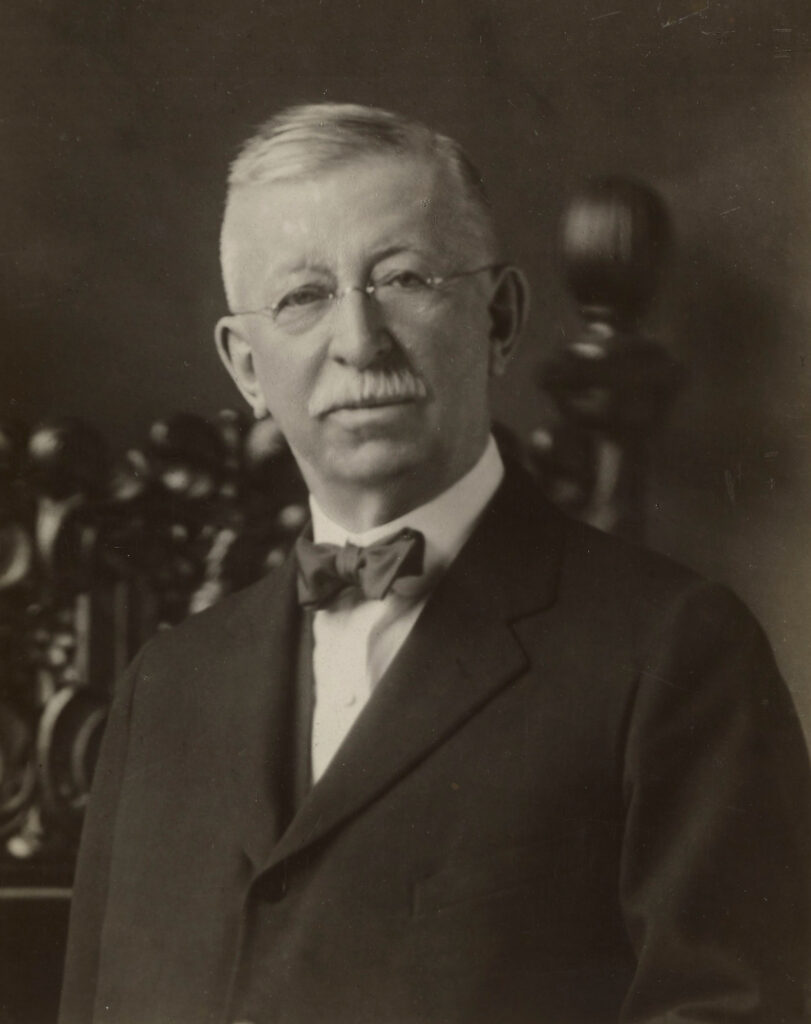
Smith, to his credit, was successful in his pursuit. In building his pantheon of American chemists, Smith helped establish the University of Pennsylvania as a leading collector and place to study the history of chemistry, which eventually contributed to the creation of the Chemical Heritage Foundation, now the Science History Institute, by Penn professor Arnold Thackray.
After Smith died in 1928, science pretty much forgot about Carey Lea, his work mostly remembered by historians of photography.
But in 2003 Laszlo Takacs, a metallurgist and physics professor at the University of Maryland, Baltimore County, published “M. Carey Lea, the Father of Mechanochemistry” in the Bulletin for the History of Chemistry. “Lea’s results on the decomposition of some compounds by mechanical action are still the clearest demonstrations of the fact that the chemical changes produced by mechanical action are distinctly different from those effected by heat,” Takacs declared. “These results secure for Matthew Carey Lea a place among the great chemists whose contributions are valid and important more than one hundred years after their publication.”
Takacs, who died in 2019, became the key figure in pulling together the history of mechanochemistry. He resurrected Lea’s work as well as other 19th-century investigations into the field, found traces of mechanochemistry in the writings of ancient Greek philosopher Theophrastus, and highlighted research pursued by Soviet and Eastern European scientists in the 1960s.
The apparent aim of Takacs’s studies was to make mechanochemistry identifiable as a coherent field of study—to recover mechanochemistry’s history so it could be seen as a defined scientific discipline, which it wasn’t for most of the 20th century. And like Smith, Takacs saw history as a tool for creating community. In a special 2004 issue of the Journal of Materials Science on mechanochemistry and mechanical alloying, he wrote, “It is expected that a sense of common historical roots increases the cohesion of a research community.”
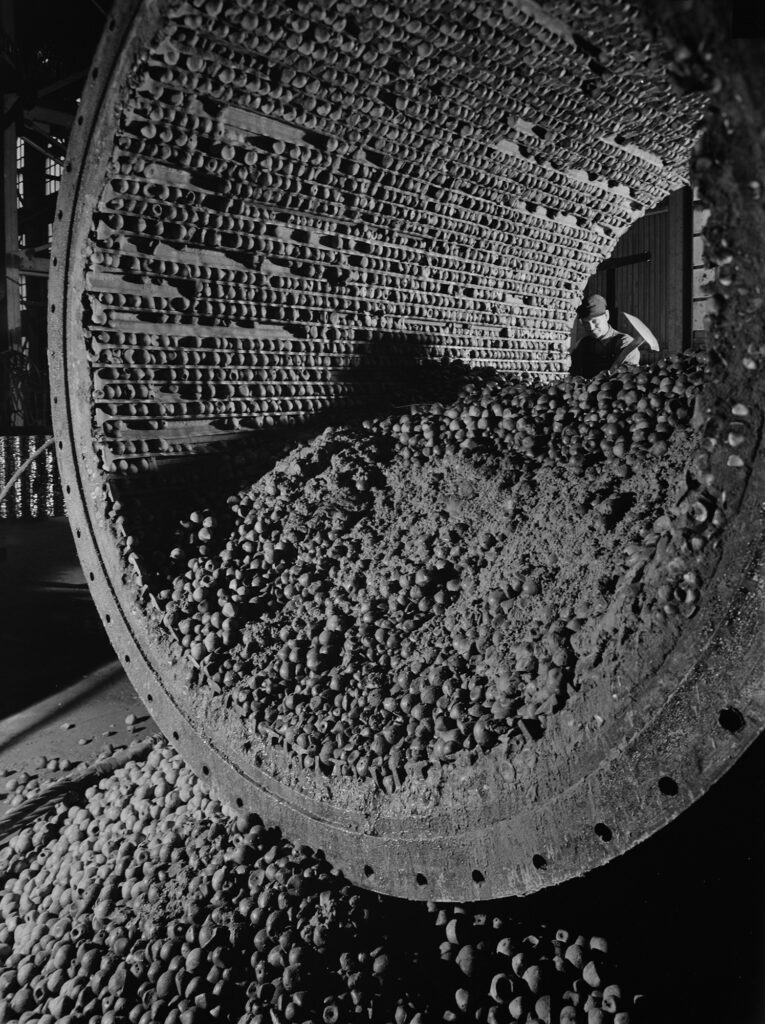
The essay you are reading is something of a third reconstruction of Carey Lea’s story, part of an effort to develop greener alternatives to the way chemistry is done today.
During the 20th century, chemists harnessed solvents and heat to develop the huge range of chemicals forming the foundation of today’s material world. They worked in a time when heat was cheap. Disposing of toxic solvents was often cheap, too, and without consequences—at least for the firms doing the disposing.
According to a Bloomberg Intelligence report, today the chemical industry is responsible for 6% of global greenhouse gas emissions, and its carbon footprint keeps growing. The industry’s dependence on solvents has also endured. Solvents make up 80% to 90% of the material used in a typical reaction and account for as much as 85% percent of the waste produced. Globally, chemical industries consume 30 million tons of solvents each year, says Tomislav Friščić, a chemist at McGill University. Business analysts expect the global solvent market to grow by more than 60% by 2028.
In the age of climate disruption the full costs and consequences of 20th-century chemical production can no longer be ignored. Many communities—often composed of poor people and people of color—have suffered from prevailing practices.
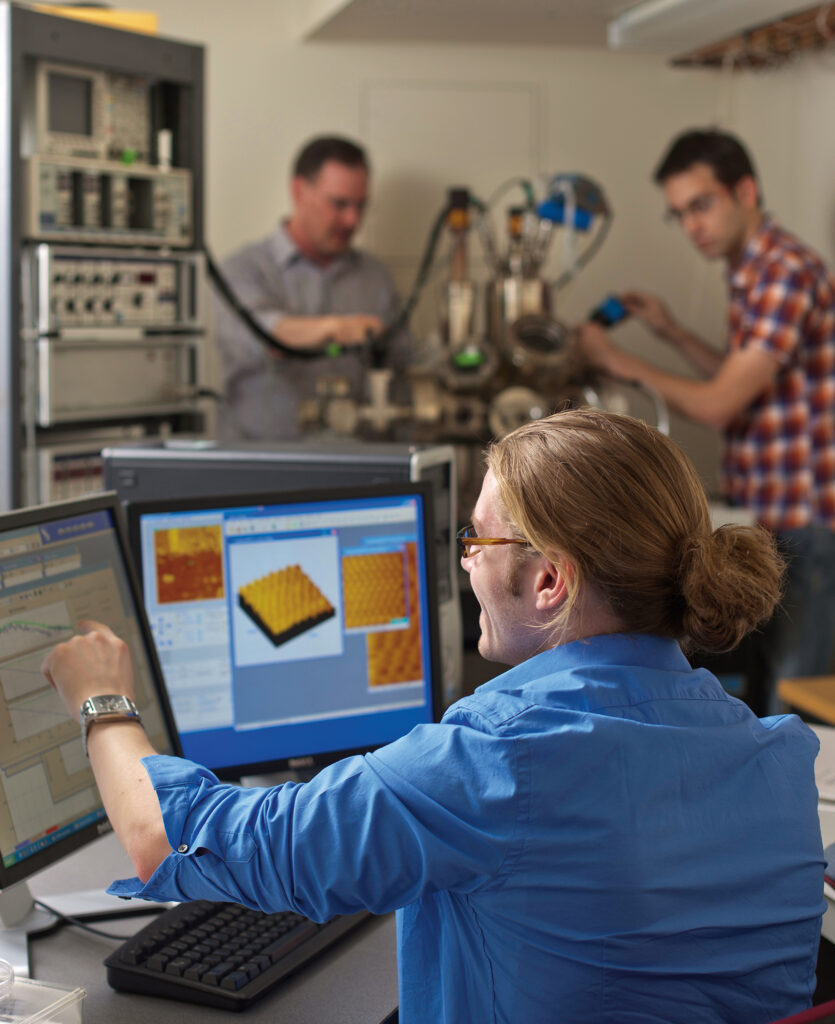
In response a rising generation of mechanochemical researchers are working to expand our understanding of the chemical reactions that occur when molecules are pressed together by mechanical forces. Advances in computer modeling and the emergence of new tools, such as atomic force microscopes, allow researchers to measure and simulate such reactions at the molecular level. Improved versions of shakers and ball mills, tools used for centuries by miners and pottery makers, can whittle down chemicals to uniform nanoparticles. The energy demands of such methods are low, just enough to spin the mills. Reactions are achieved with little or no solvents and at room temperature, making this a far greener way to synthesize chemicals and materials.
In some cases, mechanochemistry has created industrial products that were impossible to make otherwise. Beginning in the 1960s, metallurgists used ball milling to get nickel to accept oxide particles that were rejected during melting. These oxide dispersion–strengthened alloys can withstand extremely high temperatures in nuclear reactors and jet engines. Only recently have researchers discovered experimental methods for making these alloys otherwise, using lasers and 3D-printing principles.
In other cases, mechanochemists hope to replace solvent-based production with mechanical processes that require less energy and produce less waste. At an experimental level, chemists Deborah Crawford and Evelina Colacino have used an extrusion process consisting of intermeshing screws that grind together reactants to replace heat and solvents in the production of well-known muscle relaxants and antibiotics.
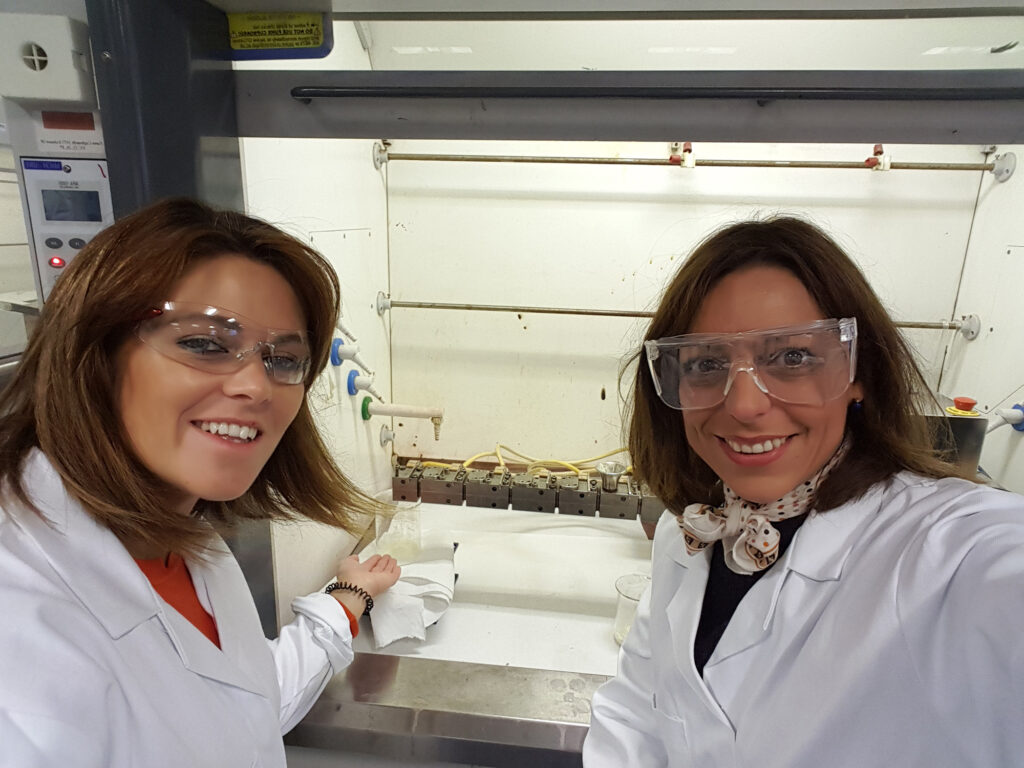
Such innovations are promising, but the historical trajectory of chemistry is powerful. As science journalist Sam Lemonick commented in Forbes in 2016, “Organic synthetic chemists—the ones who make molecules for drugs, plastics and other carbon-based things—have had almost two centuries to perfect their craft.” Solvent chemistry, despite its environmental drawbacks, works. It’s predictable and reliable. Outside of mechanical alloying, mechanochemistry remains largely experimental today.
While mechanochemistry represents a path not taken in 20th-century chemistry, it may be a vision of the future. Its methods are not intrinsically more difficult; scaling up would not require giant leaps in technology. As more people recognize the social and environmental impacts of industry and demand action to address climate change, the old ways of doing things may no longer seem practical. In that way the story of Matthew Carey Lea might point to a new vision of chemistry and its place in the world.
Funding for this article was provided by the Center for the Mechanical Control of Chemistry under NSF Grant #CHE-2023644, and Texas A&M University.