One winter day in late 1940 English policeman Albert Alexander was pruning roses in his garden. A thorn scratched his face, a common accident when working with roses. But unluckily for Alexander bacteria spread from the cut into his bloodstream, where they multiplied and started a cascade of events that standard antibacterial treatments could not stop. Abscesses grew and grew on his face and in his lungs. In a last-ditch effort to save his life, doctors at the Radcliffe Infirmary in Oxford gave him an experimental drug. It worked, and Alexander began making a swift recovery.
The miraculous substance was called penicillin, taking its name from the mold that produced it, Penicillium chrysogenum. The mold secretes the molecule and uses it as a weapon in its constant competition with other molds and bacteria. Alexander Fleming discovered the microbe-killing ability of the molecule in 1928, but the stuff proved difficult to purify in quantities large enough to be useful. Penicillin remained just an intriguing curiosity until 1939, when a group at Oxford University collected as many containers as they could find, including bathtubs and bedpans, to grow the Penicillium mold. They went on to build a small fermenter to make it easier to change out the sucrose-infused broth that nourished the mold. In 1940 they amassed enough of the drug to save lab mice infected with Streptococcus, which can cause illnesses that range from a sore throat to blood poisoning.
But bathtubs, bedpans, and the single fermenter couldn’t produce enough penicillin to save Alexander’s life. The scientists even resorted to extracting from the patient’s urine the half of the penicillin his body had not used. But it wasn’t enough. He relapsed and died a few days after the penicillin ran out. To save people the mold would have to be grown on a much larger scale, in much bigger containers, such as large beer fermenters. But species of Penicillium do not behave like beer yeast. The Oxford mold produced penicillin only when under stress, such as when crowded in shallow containers; when drifting freely in deep containers, the mold produced just a smidgen of the substance.
How to create huge quantities of sufficiently distressed mold?
Wars often act as technological and scientific accelerants. Britain was already at war, but its chemical industry was working at full capacity. The accelerant needed to turn an interesting new drug into a lifesaver would have to be found elsewhere and would require sophisticated pharmaceutical capabilities and some ad hoc gene tinkering. The United States was the only place with these capabilities.
In July 1941 penicillin research began at a Department of Agriculture laboratory in Peoria, Illinois, whose researchers had experience with fermenters and fermentation. There, scientists soon found they could increase the yield of penicillin by changing the type of sugar fed to the mold and by adding a by-product of corn milling—corn-steep liquor. But to produce enough penicillin to make a real difference, they had to find a variety of Penicillium that produced useful quantities of the drug when submerged in the broth. The lab asked for soil samples from around the world, or at least from those areas still under Allied control, and tested them for different strains of Penicillium mold. The best strain of all was found close to home, on a moldy cantaloupe in Peoria. That mold was then hit with X-rays and ultraviolet radiation, which raised the penicillin’s potency.
On December 7, 1941, Japan attacked Pearl Harbor, and a day later the United States declared war. Ten days after the attack, drug companies Pfizer, Lilly, Merck, and Squibb agreed to cooperate in scaling up penicillin production, though they were unsure whether they could produce sufficient amounts to turn an experimental drug into one useful to the war effort. To succeed they would have to match a pure culture of the revved-up Penicillium with the right nutrient broth, all maintained at the right population density, temperature, oxygen and pH levels, and so on for all the various stages of fermentation, and stirred at speeds that would not damage the living cells. As Pfizer executive John Smith said, “The mold is as temperamental as an opera singer, the yields are low, the isolation murder, the purification invites disaster.” Yet by early 1944 huge quantities of penicillin were being produced in 10,000-gallon tanks.
Microbes once produced only those substances they needed as opposed to what humans wanted. Occasionally there was overlap, as with penicillin, but such finds were exceedingly rare. Things changed in the 1970s when the biotech revolution gave researchers new tools. Rather than the uncertain outside-in effects of X-rays and ultraviolet radiation on genes, scientists could now directly insert foreign DNA into a microorganism’s genome, let the organisms multiply in a fermenter, and get the products they wanted.
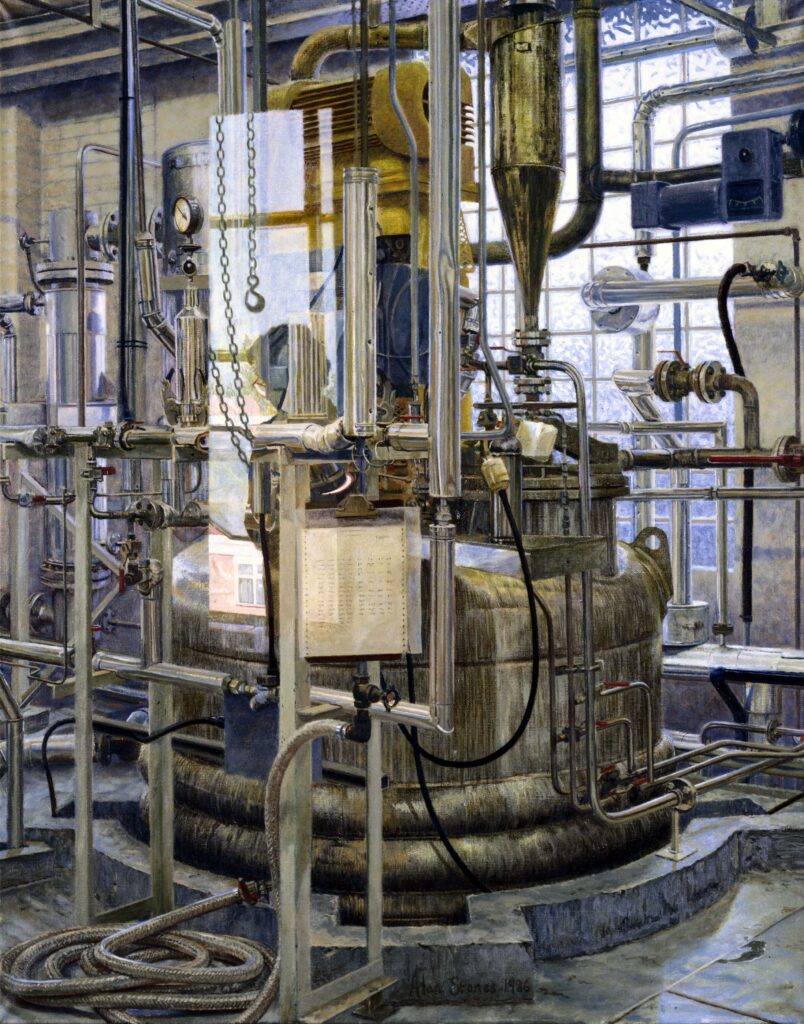
While to most people Escherichia coli is best known for the intestinal trouble it can cause, by the 1970s the bacteria was a favorite laboratory organism used to study fundamental aspects of biochemistry and molecular biology, especially the basic mechanisms of molecular genetics. Scientists at Genentech and Eli Lilly launched the biotech revolution in 1978 by genetically modifying a strain of E. coli to carry the gene for human insulin. After successful trials in 1982 the FDA approved the new insulin, named Humulin. Where diabetics once had to depend on insulin extracted from the pancreases of pigs and cattle and gamble with allergies to the medication, now they finally had insulin better suited to the human body.
A decade later the Danish company Novo (since 1989, Novo Nordisk) began to manufacture genetically engineered insulin using yeast, that venerable agent of fermentation for beer and bread. But neither Humulin nor Novo’s insulin have the exact same molecular features as human insulin: these have to be added through further chemical manipulation of the bioengineered insulin.
E. coli and yeast remain the preferred hosts for manufacturing recombinant insulin. But for many other pharmaceuticals, mammalian cells are the first choice despite the many drawbacks: they are fragile, they need to be anchored to a surface, and it takes a long time for them to double in number (18 to 24 hours compared with 45 or 90 minutes for bacteria or yeast). Their advantage lies in the accuracy with which they can express human genes. Cells taken from the ovaries of Chinese hamsters (CHO cells), having long been used in genetic, toxicity, and nutrition studies, were prime candidates for use by the emerging biopharmaceutical industry.
In 1987 Genentech brought to market the blood clot–busting drug Activase (alteplase), made using CHO cells adapted to grow in 10,000-liter fermenters. Activase was the first of a class of drugs known as tissue plasminogen activators (tPAs), which are now standard treatment for stroke, blood clots in the lung, and heart attacks.
One of the biggest differences between 1940s and present-day fermenters is the amount of automatic analysis and control made possible by computers. In living memory scientists and technicians worked with notebooks filled with readings from various gauges. Scaling up a process to commercial proportions once required scientists and engineers to sleep overnight in their labs in case adjustments had to be made. Nowadays there is a great deal of automated monitoring and adjustment, although alarms are sent to the scientists at their homes if significant modification seems in order.
In 2008 the Science History Institute (then the Chemical Heritage Foundation) acquired a fermenter from the early 2000s with these bells and whistles. Currently stashed away in a New Jersey storage building, the fermenter stands almost 6 feet high with its legs only partially extended; its double-walled tank is made of special stainless steel and can hold 150 liters of liquid. With its computerized analyzer and controls, it weighs about a ton. The pumps that would have supplied sterile air, water, nutrients, carbon dioxide, and antifoaming agents lie in a jumble in a large carton next to the fermenter. Originally the whole setup was assembled by B. Braun Biotech USA (now Sartorius Stedim Biotech) and was in service at Centocor Biotech (now Janssen Biotech) from about 2002 to 2006.
In its working life the fermenter would have stood in a glassed-in sterile chamber, connected to larger fermenters and a series of similarly protected equipment that isolated the desired product from the mixture of micro-organisms, by-products, and nutrient broth. Staff members preparing inoculations of micro-organisms for fermenters or retrieving samples of the brew in progress had to enter the aseptic chamber via airlocks between the relatively high-pressure area in which the fermenter stood and the rest of the facility. Like doctors and nurses in an operating room, these workers would have been garbed tip-to-toe in white or pastel outfits.
The Institute’s fermenter was used to produce monoclonal antibodies (MAbs). Genetically engineered MAbs, Centocor’s specialty, are now a decades-old technology but are still among the most cutting-edge products of fermentation. MAbs mimic and improve on one of the body’s chief means of combating disease and restoring health: antibodies. The first MAbs were made by fusing mouse antibody-producing cells with mouse tumor cells; the fused cells went on to churn out antibodies thanks to their quick reproduction, a consequence of their cancerous heritage. The antibodies produced by cloning of a single antibody are identical, hence monoclonal, and more efficient than the body’s own immune system, which responds by producing a variety of antibodies, some useful and others not. The first drug produced in this way was Orthoclone (muromonab-CD3), designed to stop the immune system from rejecting a transplanted kidney. The drug was approved for use in 1985 but was later superseded by mouse-free MAbs that did not induce allergic reactions in patients.
Starting in 2002 the Institute’s fermenter used CHO cells to manufacture human MAbs for phase I clinical trials, in which healthy people are tested to establish safe dosage levels, and phase II trials, in which the drug is tested on 100 to 200 people sick with the disease being targeted. These tests determine the drug’s safety and efficacy. (Because of the fermenter’s relatively small capacity it was not used in phase III trials, which typically deal with 300 to 3,000 or more patients.) These trials resulted in two drugs. One, Stelara (ustekinumab), is used to treat plaque psoriasis, a skin disease in which patients suffer from red, itchy patches and silvery scales and that sometimes leads to arthritis. The other, Simponi (golimumab), is also used to treat psoriasis and to alleviate ulcerative colitis.
Microbes once produced only those substances they needed as opposed to what humans wanted. . . . Things changed in the 1970s when the biotech revolution gave researchers new tools.”
Another success connected with the Institute’s fermenter is Sylvant (siltuximab), a drug used to treat multicentric Castleman disease, a rare and often fatal blood disorder in which the body overproduces lymphocytes, a type of white blood cell. The condition causes lymph nodes and lymphoid tissue all over the body to swell, including in the lungs, stomach, and other organs.
Since the fermenter had such a remarkable track record, why would Centocor have given it away? The decision to decommission it was probably based on a combination of factors. Its internal surfaces may have developed scratches or stubborn deposits that could harbor contaminants, or it may have become mechanically or electrically unreliable. Perhaps more-advanced models came on the market, offering more sophisticated computer analysis and control capabilities.
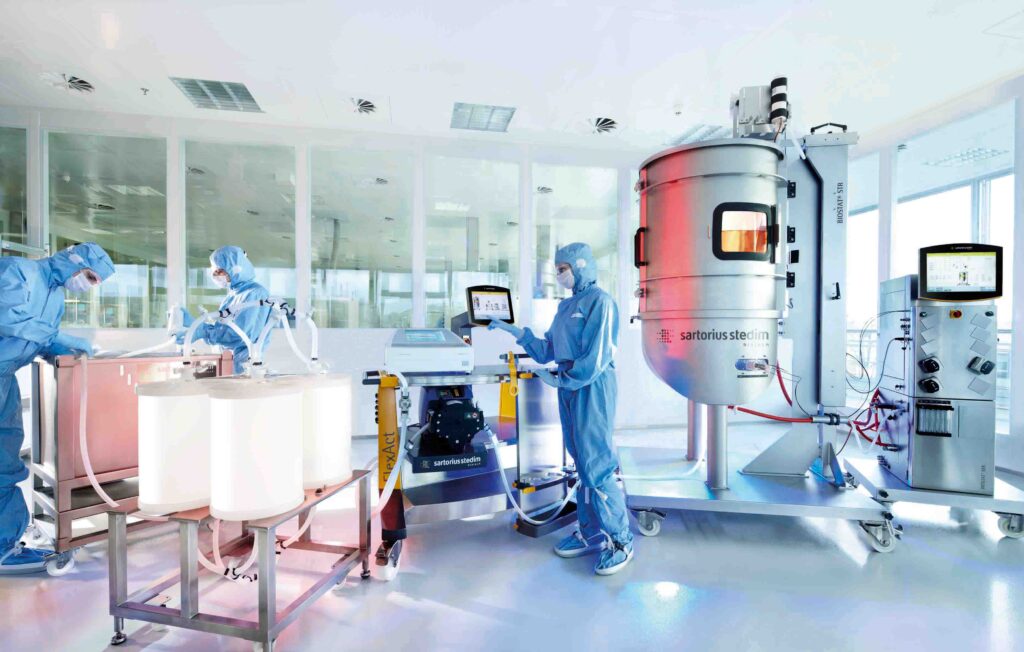
Although fermenters such as ours appear regularly on the used fermenter market—eBay does a surprisingly brisk trade in lab-grade and commercial equipment—some biotech firms have been donating usable equipment to universities and showpieces to museums and collections. That might be because the days of small-scale fermentation using only double-walled steel tanks are coming to an end. Increasingly, small-batch runs are being made in disposable fermenters composed of layers of flexible plastics, which can be thrown away when the run is over.
This next generation of fermenters is brewing up more and more MAbs, with a steady stream of drugs that target more common afflictions. In 2014 Merck’s Keytruda (pembrolizumab) was approved for use in treating melanoma, the deadliest type of skin cancer, and a year later was approved to treat the most common lung cancer, non–small cell lung carcinoma. Future breakthroughs in pharmaceuticals will, like penicillin and MAbs, owe their existence to evolving biological sciences teamed with advances in fermentation technology.