Icy Beginnings
In 1884 a dispatch in Science relayed an odd discovery by Swiss scientist François-Alphonse Forel. While climbing a glacier in the Bernese Alps, Forel came across broken boulders inscribed with faint dates and hard-to-read names. He soon realized these were the scattered remains of a crude mountaineering shelter that had stood a few miles away, nicknamed Hôtel des Neuchâtelois by the pioneering circle of glaciologists who had camped in it 40 years earlier and signed their names on its stones as they might a guestbook.
That the legendary lodging—where early ideas in glaciology crystalized—was ripped apart by the drift of glaciers was an apt metaphor for the schism that divided alpine researchers during the mid-1800s.
In 1840 Swiss geologist Louis Agassiz published Études sur les glaciers. In it he proposed the controversial idea of an ice age—a time in which sheets of ice had covered the northern hemisphere, gouging out the landscape as they moved. Through an exhaustive study of fish fossils, Agassiz had convincingly resurrected the deep past before; nevertheless, his glacial theory was widely dismissed by scientists who believed the Bible’s great flood had eroded Europe’s gorges and scattered boulders across the continent.
To win support for his theory, Agassiz invited scientists to visit his alpine research station and see the evidence for themselves. Scottish physicist James Forbes came to the Hôtel des Neuchâtelois in 1841. While there, Forbes noticed curving blue striations in a glacial crevasse.
Agassiz dismissed the observation, suggesting the distorted lines were just light playing off layers of sand that had dusted the glacier as it formed. But Forbes found more striations, ultimately deducing the twisted lines showed the icy behemoths were viscous—with pressure inside the glaciers causing them to fold in on themselves as they moved. When the Scotsman published his theory in an 1843 book alongside a note of surprise that Agassiz had paid the evidence no mind, Agassiz was furious.
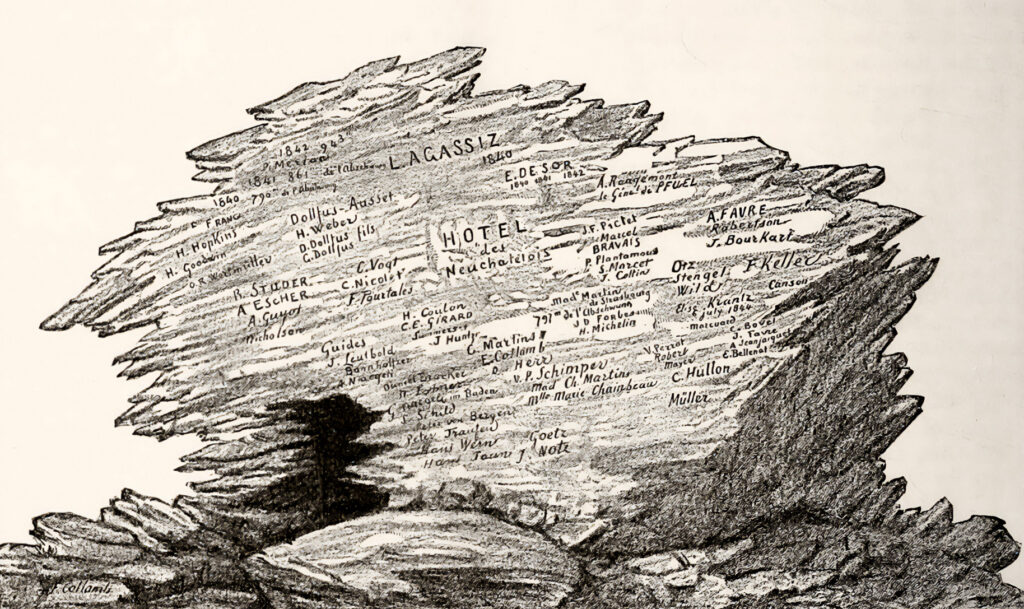
Agassiz sent off vitriolic letters to 500 scientists blasting Forbes. In them he asserted that the striations were a feature previously observed by other glaciologists and that he had planned to write about them later, implying Forbes wasn’t giving due credit. The back and forth went on for nearly 15 years.
Then up-and-coming Irish scientist John Tyndall jumped into the fray, saying Forbes was all wrong.
Drawing on Michael Faraday’s “regelation” experiments, Tyndall asserted that the force propelling glaciers was not viscosity but the breaking of ice under the pressure of its own weight and subsequent refreezing when pressure reduced. If a glacier was viscous and plastic, he opined, it would not crack and abrade when it passed through valleys.
Tyndall, a masterful showman, publicly pooh-poohed Forbes and his theory, bragging in a letter that he “so utterly annihilated it that its author will hardly acknowledge it.” He went so far as to accuse Forbes of plagiarizing the theory from recently deceased glaciologist Louis Rendu (who had made no such complaint while alive). The takedown continued for more than a decade. By the end of it, as one ally cheered, Tyndall had “run an oiled rapier” through Forbes.
The beleaguered Scot, who maintained that the clashing theories were in fact complementary, would have agreed.
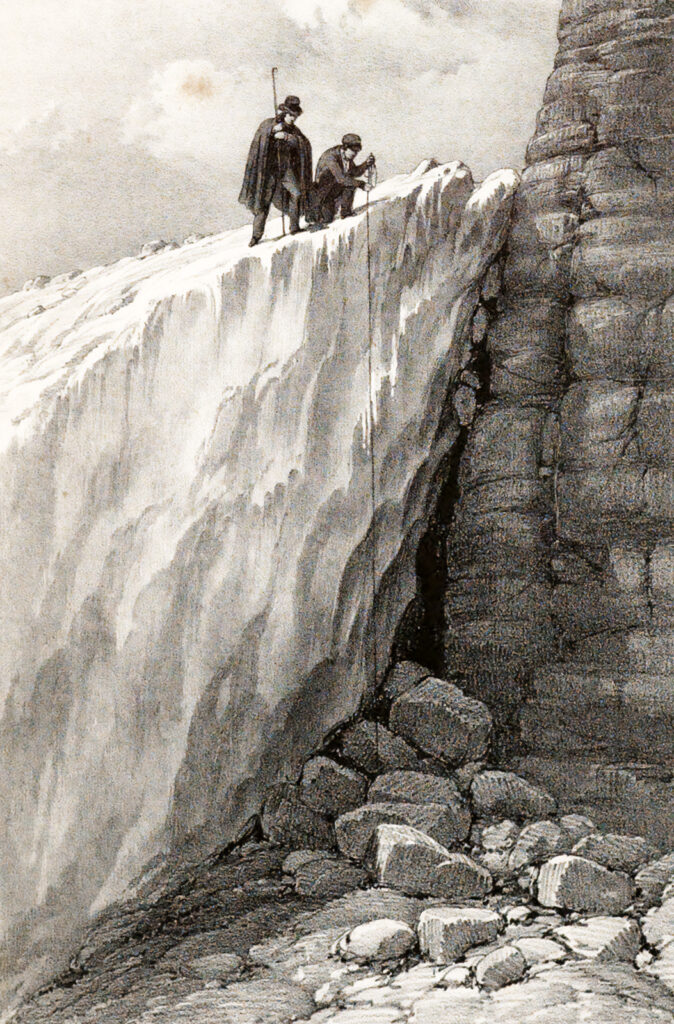
‘‘For about three years I have felt disheartened and hopeless as to stemming the popular tide of propositions against my Theory of Glaciers—or I should say against me personally as its author,” he wrote to one of his remaining scientific allies in 1864. Four years later Forbes died at age 54, a man broken by the vicious attacks on his ideas about viscosity.
By the time Forel’s report ran in Science, Tyndall was the only combatant still living, and his ideas were carrying the day. Scientific interest in glaciers, in no small part fueled by these controversies, had heated up even more in the 1880s. In Belgium, a physicist and experimental chemist named Walthère Spring was taking the glaciologists’ theories in a new direction, picking up on the force that played a crucial role in all three of their models—pressure.
An avid mountaineer himself, Spring was fascinated by pressure, a force so tremendous it could drive glaciers across continents and cut valleys out of mountains. What else, he wondered, could such tremendous pressure do? If the weight of glaciers could squeeze snowflakes into metamorphic rock, what could the crush of a sliding glacier do to the minerals inside the earth’s crust?
Pursuing this curiosity would lead Spring to the precipice of an entirely new scientific field and send him tumbling into a scientific dispute that echoed those of the generation before.
A Curious Man
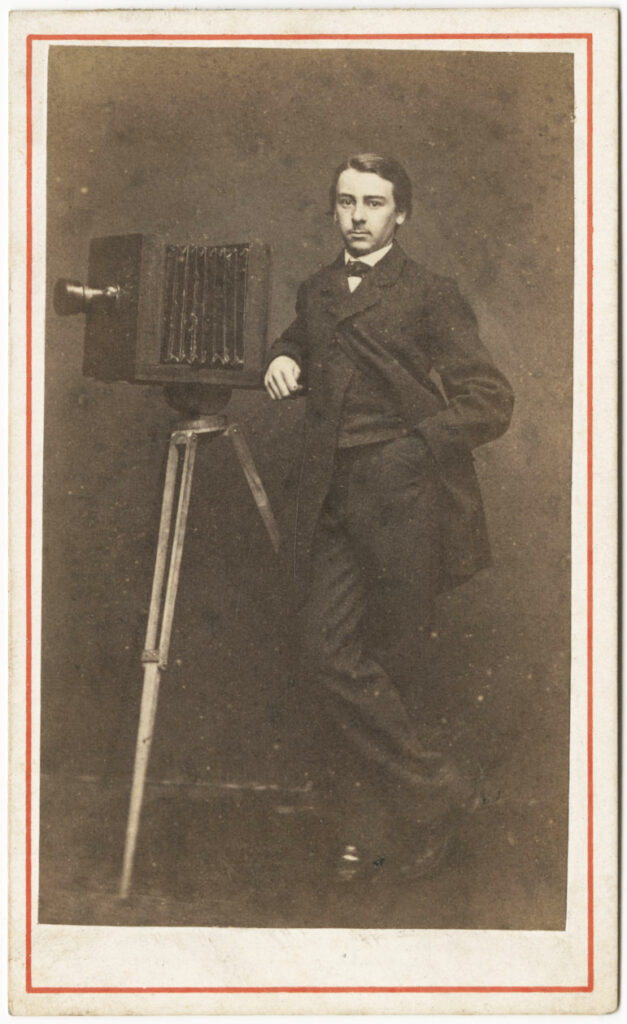
Young Walthère Spring didn’t show much early aptitude for academia, a disquieting trait for his father, a distinguished professor of physiology at the University of Liège. The shy boy preferred tinkering and inventing things, from elaborate locks to cameras. His struggles with Greek and Latin led to failing marks on his university entrance exams. Instead, he found work as a gunsmith at a metallurgical firm, where he refined his talent for fabrication.
With the encouragement of his influential godfather, chemist Jean Stas, Spring retook the exams, passed, and entered the School of Mines at the University of Liège, where he excelled. Six years later he packed off for Bonn, Germany, to study physics and chemistry with such eminent scientists as Rudolf Clausius and August Kekulé. Under Kekulé’s mentorship, Spring undertook research into classes of sulfur compounds known as polythionates and sulfur oxoacids, discovering new chemical reactions and synthesizing novel compounds.
In 1876 he was hired as a professor of chemistry at the University of Liège. A year later, at just 29 years of age, he was made a corresponding member of the Académie royale des Sciences, des Lettres et des Beaux-Arts de Belgique. He opened the University of Liège’s first teaching lab in 1880 and became known for impassioned lectures on the beauty of hypothetical thinking.
“His main message was that science should be open to new facts, new hypotheses—especially in a young field such as organic chemistry,” says historian of science Brigitte Van Tiggelen. “A science where there is no contestation, no place for a plurality of hypotheses, is actually in danger of stagnation.”
Away from the classroom he hatched experiments of remarkable variety, investigating everything from the cause of color blindness to mercury’s electrical charge. Spring was still very much the tinkerer, eschewing research with practical application for basic science and experiments into minerals and geology and whatever else struck his fancy.
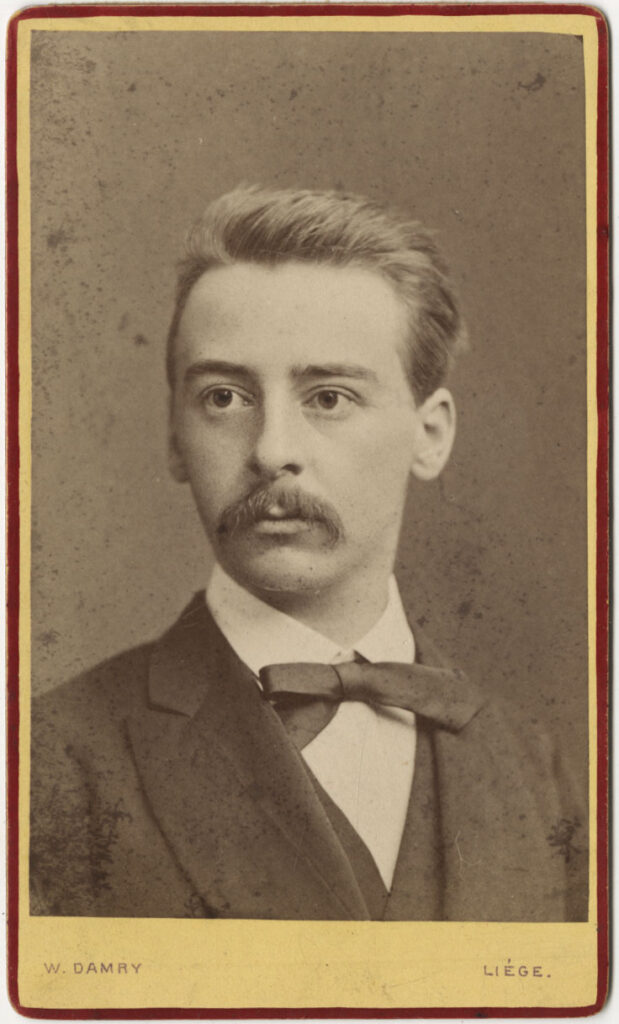
“He becomes a professor at exactly the time when freedom in academic research is considered a virtue,” says Van Tiggelen. “He’s [eventually] given an impressive building for his laboratory. And since he was at a state university, there was a lot of money. . . . He’s not an outsider, but he’s following his own track, his own pursuits. In that sense he was more of a lonesome cowboy.”
Spring, never a very social creature, struggled to get along with his colleagues at the university and spent much of his time outside of the classroom alone in his laboratory, pairing his gift for device building with a ceaseless curiosity. Inspired by Tyndall’s 1870 work postulating why the sky appeared blue, Spring constructed an elaborate apparatus from 85 feet of glass tubing to successfully demonstrate that the color of pure water is actually cerulean.
He was meticulous in his observations of the world.
At the same hour every day for a year, he and a collaborator studied the Meuse River, analyzing the color and composition of its water. In 1884, the year Spring was made a full member of the Académie, they co-published their findings, including calculations of the river’s flow and amount of sediment transported annually. The following year he co-published a paper showing that coal-powered Liège was warmer than outlying areas and attributed the fact to higher emissions of carbon dioxide in the city. In this early observation of the greenhouse effect, the authors even landed on the now familiar metaphor.
“The atmosphere, charged with water vapor and CO2, protects the earth against a cooling as does a greenhouse,” they wrote.
Mimicking Glaciers
Spring’s most innovative—and controversial—research focused on pressure, which he began publishing in 1878. He wanted to know exactly how the weight of glaciers affected ice—as well as the rocks and minerals crushed underneath them—by trying to replicate glacial pressure in his laboratory.
“The peculiarity of experimental science, especially in the 19th century, is that you observe something in the natural world, and if you want to understand the phenomenon, you have to reproduce it in the laboratory where you can [control] the process,” says Van Tiggelen. “And that’s why he comes to the idea of having this press. It’s kind of a metaphor for the glaciers applying pressure on rocks. But he has a wandering mind. So once he starts, he thinks, ‘Oh, yeah, but what else could I do with this?’ ”
Did Tyndall’s idea of cracking and regelation apply to more than ice? If Spring pressed other solid materials under the right conditions, would they too meld and form solid blocks? Could pressure create “artificial minerals”?
To test his questions, Spring first used a screw press, but realizing it had shortcomings, designed a more elaborate device using a piston, weights, and a vacuum pump to keep atmospheric gases from influencing his experiments. The end result was a massive, lever-operated mechanical press that could deliver immense pressures on samples—greater than 25,000 atmospheres, by some estimates. Spring was careful to keep most experiments under 7,000 atmospheres to avoid deforming the piston, but those levels still approximated geological conditions. To measure the effects of time, he kept some samples under pressure for 17 years.
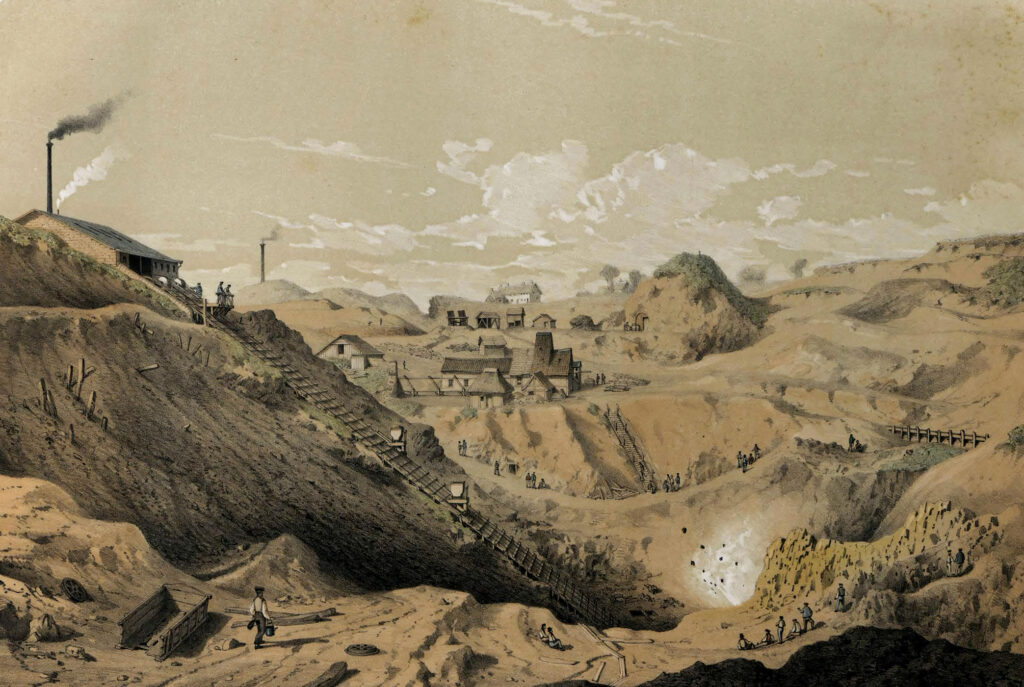
He soon found that his press could weld metal powders into compact blocks. Polished cubes of copper and zinc pressed together came out joined by a layer of brass. He showed that under pressure peat converted to what appeared to be lignite (soft coal), that chalk turned marble-like, and layers of clay pressed together with organic material produced lumps of schist that looked like the rocks scattered across the Belgian terrain.
What’s more, he found that some materials would chemically react under pressure. He demonstrated, for instance, that compressing barium sulfate and sodium carbonate over time would produce barium carbonate and sodium sulfate.
Achieving these reactions was demanding work. Lazlo Takacs, a physicist who studied Spring’s career, recounted the process.
“First the starting powder mixture was pressed into a pellet, then filed to obtain a more uniform powder, compressed again into a pellet, filed again, etc. until the final product was obtained.” The fussiness of this method, Takacs notes, created a problem for Spring. “Unfortunately, the conditions were very complicated, thus it was difficult to identify the exact cause of the reactions.”
Cracks Appear
After Spring published a paper in 1881 declaring that some powdered metals could be compressed into crystalline form, a group of researchers in Paris cried foul, claiming they had tried to replicate his experiments but concluded in 1883 “that pressure alone is not enough to crystallize bodies.”
Spring protested that the materials they used were impure and that they hadn’t performed the experiments in a vacuum as he had. The criticism so irritated Spring that he schlepped his unwieldy press to Paris to recreate his experiments before his critics and a panel of intermediaries.
According to scientist Léon Crismer, who was tapped by the Belgian Chemical Society to write a memorial for Spring, “the rebuttal of the critics was decisive.” According to other reports, some weren’t convinced. Chemist Charles Friedel, who oversaw the demonstration, wrote, “in certain cases in which Spring sees a crystalline condition and where indeed evidences of crystallization may be detected with a microscope, I cannot admit that there has been crystallization as a direct consequence of the compression.”
Ultimately, the dispute was left unresolved. “Although Spring’s rebuttal seemed more than satisfactory,” Takacs wrote, “the incident was widely reported and raised lingering doubt over the validity of his results.”
Complicating matters further, Spring’s compressor was dogged by a leak around the piston. This fact raised further skepticism about his observations.
Spring’s experiments showing that pressure could cause the welding of some metals were questioned, with skeptics asserting that the shearing action of material squeezing past the piston might create enough heat to produce the fusion he reported.
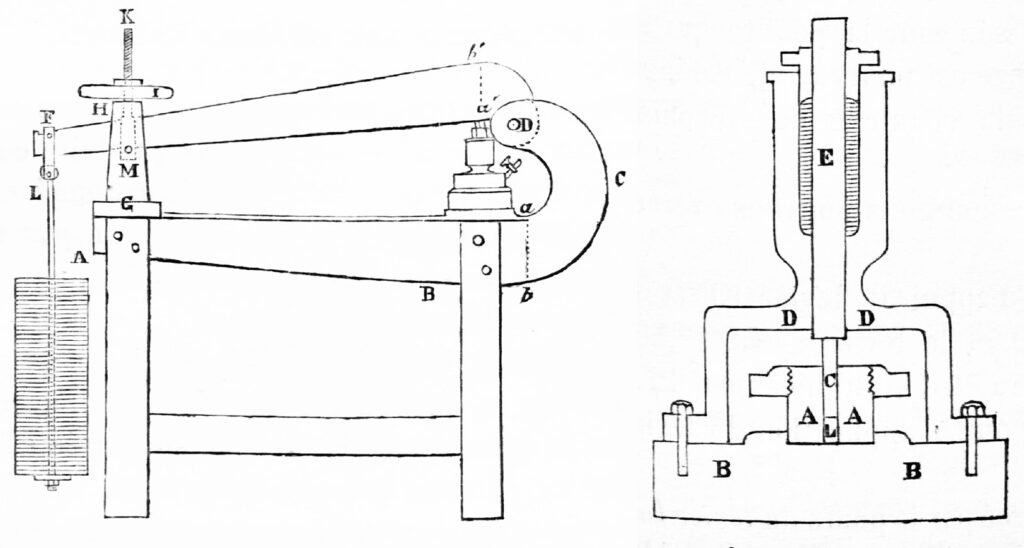
To disprove temperature played any role at all, Spring packed his press with a substance called phorone, which melts at 82°F, and placed a lead ball on top. If shearing produced enough heat to weld metal, then the phorone would quickly melt, and the lead ball would drop to the bottom of the cylinder.
“This did not happen,” Crismer notes dryly.
Spring pressed on, but the more his work was questioned, the more embittered he became.
In 1887 a paper about the behavior of solids under pressure was challenged, this time by geologist William Hallock of the U.S. Geological Survey. Invoking Spring’s previous critics, the American wrote a response in the USGS Bulletin disputing Spring’s paper, which Hallock interpreted as stating that under pressure solids could liquefy, pointing out that solids don’t melt under pressure alone. (Pressure’s exact effects on ice, which does melt under pressure alone, would be a matter of dispute for 130 years more).
In a flurry of heated letters to the Bulletin, Spring argued that his report, written in French, had been misunderstood. For instance, Hallock had translated the word for “to weld” as “to melt.” Spring also pointed out that he hadn’t said all powdered solids melted, but that some powdered solids under pressure might flow like a liquid and be welded together.
“It is quite evident,” he concluded in one response, “that there is no reason for arguing with Mr. Hallock, since his study, which rests on a chimera, is, in my opinion, null and void. But I think I have a right to protest against the carelessness which has led him to thus misstate my views.”
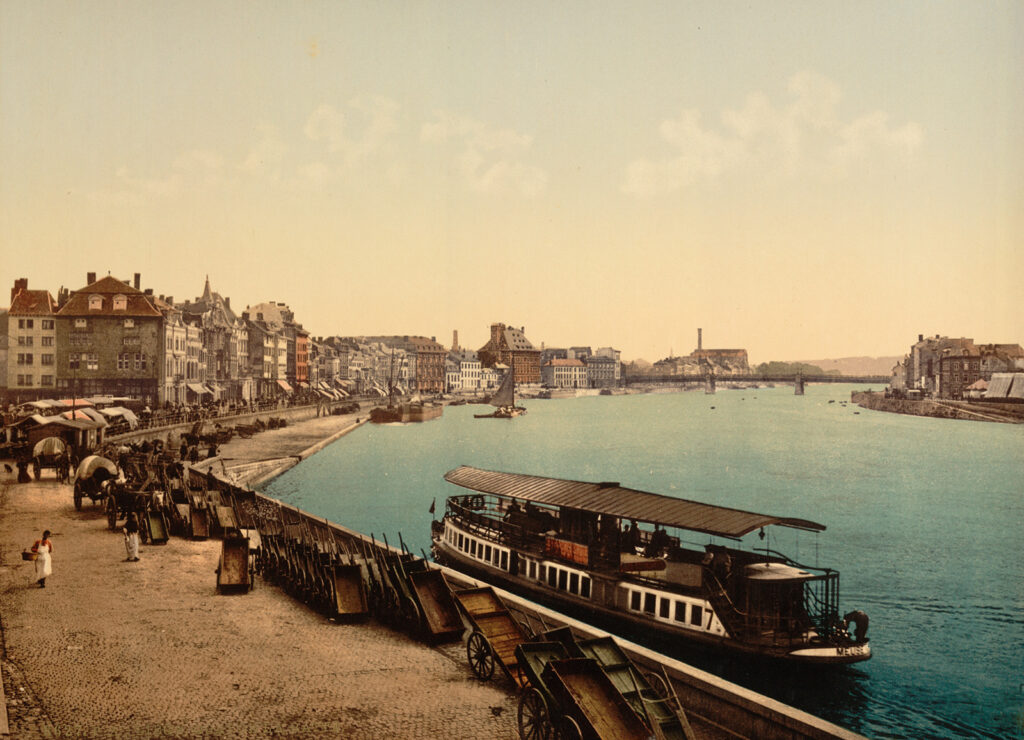
In 1894, Spring felt assaulted again by a different American. In this case the offender was a “gentleman scientist” from Philadelphia named M. Carey Lea, who like Spring found that mechanical force—without heat, light, or solvents—could bring about chemical reactions. Lea, however, had come to his conclusions via photography: he had noticed that, when jostled, certain light-sensitive silver solutions changed color. This discovery launched the American into series of experiments into the effects of force, including pressure, on silver salts and colloids.
Lea was at least somewhat aware of Spring’s research, citing his work in an 1893 paper published in a German chemistry journal. However, the next year Lea published another article in the same journal, about the chemical effects of grinding in endothermic reactions, and did not mention Spring at all. Though Spring had only touched on the matter, he assailed and shamed his perceived interloper.
“If my plan will be executed by another researcher, like Mr. Carey Lea, his effort will certainly advance science. But whether I must lose all claims to my existing results, I leave for the judgment of my distinguished colleagues.”
Shocked, Lea countered that his aims, research, and conclusions differed from Spring’s, but the Belgian continued to huff. Lea published only one more report on the matter before dying in 1897; by that time, a resentful Spring had drifted away from the pressure experiments that had so consumed him for two decades.
The Crushing Effects
Lost in all this bickering was just how pioneering Spring’s investigations were. Both Spring and Lea raised questions and conducted experiments that had the hallmark of a new chemical discipline, mechanochemistry, which would emerge in the decades that followed. Mechanochemistry looked to mechanical forces—crushing, grinding, shaking—to alter substances and elicit chemical reactions.
A few recognized Spring’s contributions to the young field early on. In his 1912 remembrance, Crismer praises him for marrying geology and mechanochemistry with “patience, ingenuity, skill, and experimental integrity.”
“He surely is convinced that he is opening new perspectives—like taking a new pair of glasses to observe the universe,” says Van Tiggelen. “He really wants to push things further and get into the heart of the matter with new instruments, new ways of doing things.”
But Spring never realized he was on the cusp of a new field. And despite publishing 25 papers on pressure’s ability to induce physical and chemical changes, Spring downplayed its importance all the while, writing that “one must not lose sight of the fact that pressure is not a chemical agent to the same extent as heat or electricity.”
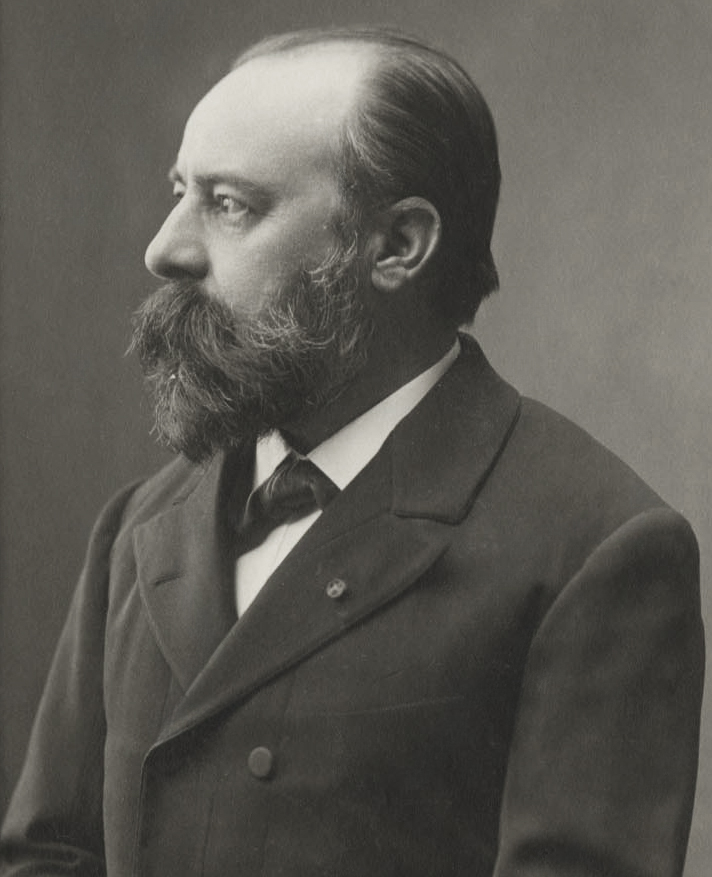
Perhaps this myopia is partly to blame for Spring’s relative obscurity in the history of science. His retiring nature, a trait he shared with Lea, may have contributed as well. These innovators inside the laboratory simply didn’t give much attention to the game outside of it.
“He was abundantly recognized in his time, but he didn’t have a school of apprentices working in his lab—postdocs and doctoral students who followed him—ensuring that his legacy continued,” says Van Tiggelen. “He didn’t have a school of students to celebrate what he had done.”
So as with Forbes, Spring’s sudden death in 1911 at age 63 left the legacy of his pressure experiments in the hands of his critics.
In 1913 American chemists John Johnston and L. H. Adams published a long review of his pressure work in the American Journal of Science. While acknowledging Spring had conducted the first systematic experiments on the effects of high pressure on solids, they asserted that his compression device delivered unequal pressure, casting a long shadow on his machine, his assumptions, and his findings. As Takacs put it, “one after the other, they showed that his methods were flawed and his conclusions incorrect.”
Most detrimental to Spring’s legacy is arguably the fate of the field he helped spawn. Outside of the Soviet Union, mechanochemistry was largely ignored during the 20th century, pushed aside by a chemical industry already reliant on solvents and heat in its production lines.
Today, with concerns about the toxicity and adverse environmental effects of chemical solvents, there’s renewed interest in mechanochemistry worldwide to produce pharmaceuticals, battery materials, pigments, and many other substances with techniques that are safer and cleaner, and have fewer energy demands.
In weighing Spring’s legacy, Takacs wrote, “His questions were revolutionary and the breadth of his studies unparalleled in the 1880s. The validity of his conclusions is a different matter.” Some of Lea’s conclusions have also been questioned. But that’s the nature of science—hypotheses floated, only to be shot down, sometimes vehemently. Methods picked apart. Even if not all of their ideas were rock solid, a foundation was laid.
It’s the same for the early glaciologists. Modern science doesn’t hold Agassiz, Forbes, or Tyndall as entirely correct in their theories either.
Agassiz was right about the ice age, but wrong about the extent of land once covered in ice as well as the speed at which glaciers move. He also missed that there had been more than one such ice epoch.
Forbes was correct that glaciers are viscous and deform due to internal pressure, but he overlooked cracking, refreezing, and other factors integral to their movement, such as meltwater and slippage.
And Tyndall’s ideas were correct when it came to glacier shattering and regelation, especially as the icy masses push against stone and through narrow passages. However, even though he won the heated dispute in his day, his chipping and refreezing model is not the primary mechanism of glacial motion, as he claimed. His ideas were in fact complementary to those of Forbes—just as Forbes had said all along.